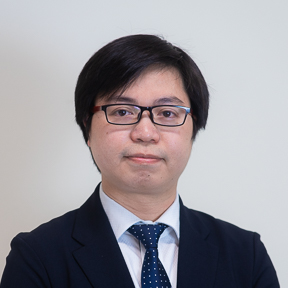
LE DUC ANH Associate Professor
Hongo Campus
Graduate SchoolGraduate School of Engineering - Electrical Engineering and Information Systems
DepartmentDepartment of Electrical and Electronic Engineering
Nano Physics & Device Technology Field
Nanostructural physics
Nanomaterials engineering
Applied materials
Crystal engineering
Thin film/Surface and interfacial physical properties
General applied physics
Quantum Computing
Semiconductor
Condensed Matter Physics
Semiconductor Process Technology
Realization of semiconductor materials and devices that integrate "magnetism", "superconductivity" and "topology".
Our focus is to integrate "magnetism", "superconductivity" and "topology" to all-in-one semiconductor platforms, using nanoscale semiconductor/ferromagnet/superconductor hybrid structures. These integrated material platforms would pave new ways to fundamental technologies for ultra-low power-consumption electronics and fault-tolerant quantum information.
Research field 1
Semiconductor Spintronics: To make semiconductors ferromagnetic
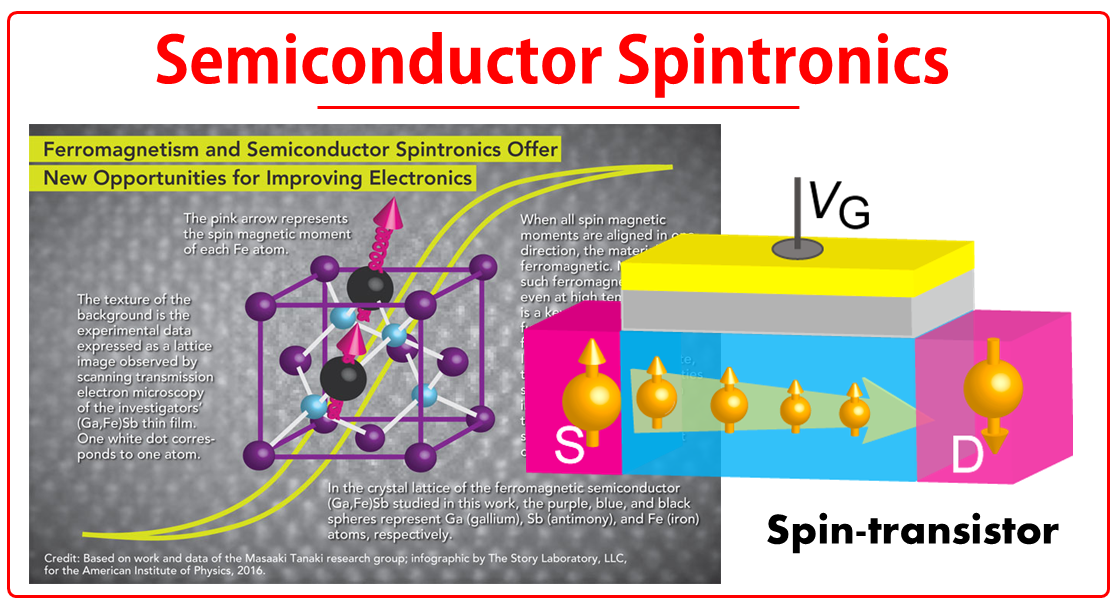
If a semiconductor can be made ferromagnetic, output state of the electronic devices using such ferromagnetic semiconductors (FMS) can be controlled by the magnetization direction, which can maintain without the necessity of supplying an external power supply (namely, “non-volatility”). Therefore, it is expected that various new functionalities such as “non-volatility” and "reconfigurability" (the ability to rewrite the circuit function by controlling the magnetization state) can be realized. By utilizing both the "charge" and "spin" degrees of freedom of electrons in semiconductors, we aim to realize new spin-based devices such as ultra-high-speed non-volatile memory, reconfigurable logic circuits, and flexible information processing, which may lead to the creation of next-generation electronics.
It is not easy to make semiconductors ferromagnetic. Our methods are by substituting some atoms of the non-magnetic semiconductors with magnetic atoms, or by producing high-quality heterojunctions of semiconductors / ferromagnets. These require utilizing state-of-the-art crystal growth technology. By adding Fe to narrow-gap semiconductors (InAs, GaSb, InSb, etc.), we have successfully realized both P-type and N-type ferromagnetic semiconductors that have ferromagnetism above room temperature for the first time. We are studying epitaxial growth, spin-related properties of these unique new materials, and their potential applications to low-power-consumption electronic devices such as spin transistors.
Research field 2
Superconductor/Ferromagnetic Semiconductor Hybrid Structures: Searching for Majorana on magnetic semiconductors
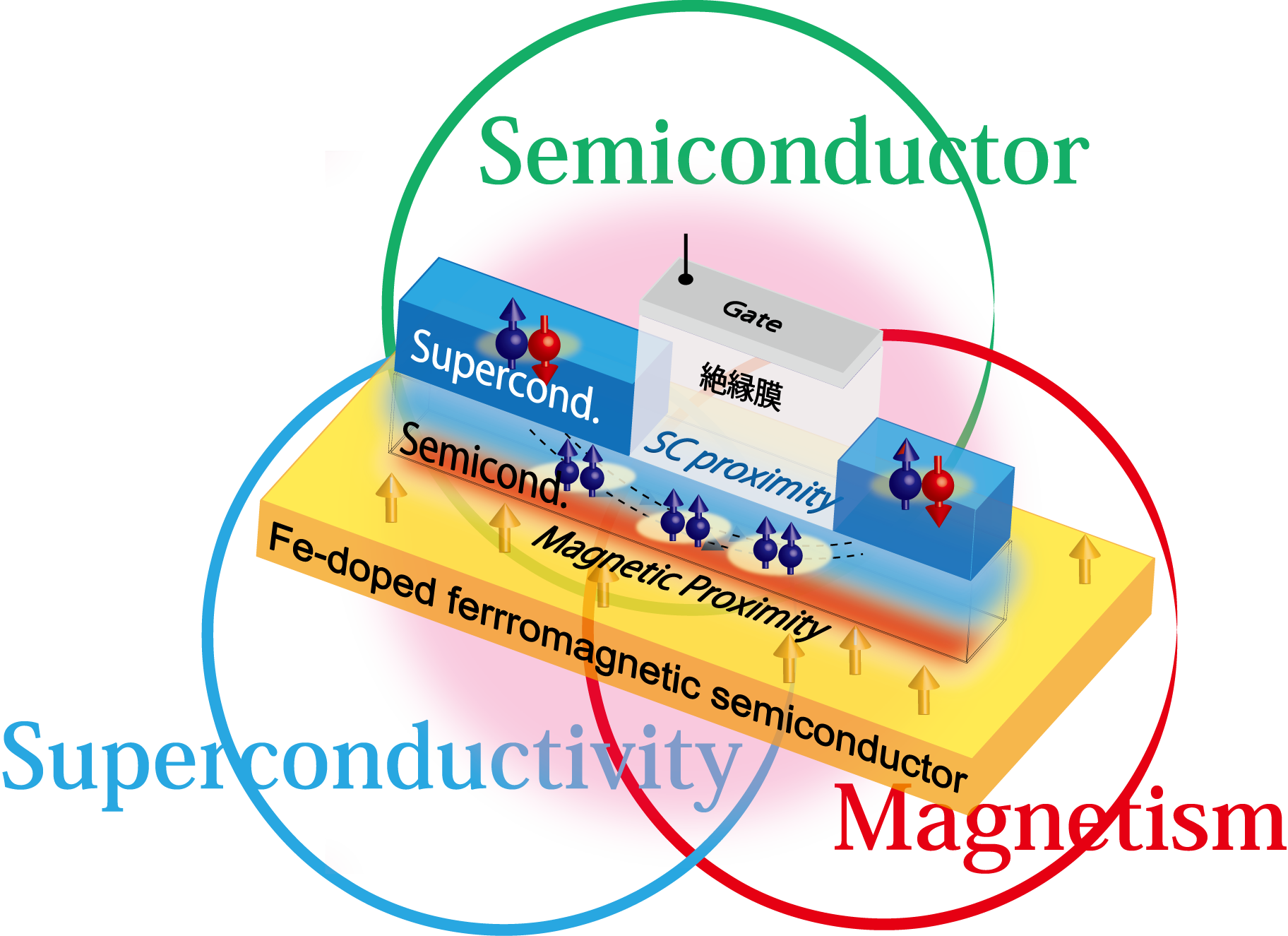
Majorana fermion (MF), which is an exotic topological state in condense matters, is highly expected to be applied to fault-tolerance topological quantum computation. MF is predicted to exist at the edge of topological superconductors, which can be realized by combining three recipients of (1) superconductivity, (2) spin-orbit interaction (SOI), and (3) spin splitting. Furthermore, future large-scale quantum computation using the MF states demands a material platform with excellent controllability and compatibility to existing technologies.
In this research, using our unique capability of crystal growth, we aim to integrate "superconductivity", "ferromagnetism" and "topology" into a single semiconductor material using ferromagnetic semiconductor and superconductor hybrid structures. These semiconductor-based topological platforms are promising for realizing MFs and studying their related topological physics.
Research field 3
Exploring new topological materials: Expanding the horizon of Dirac materials and physics
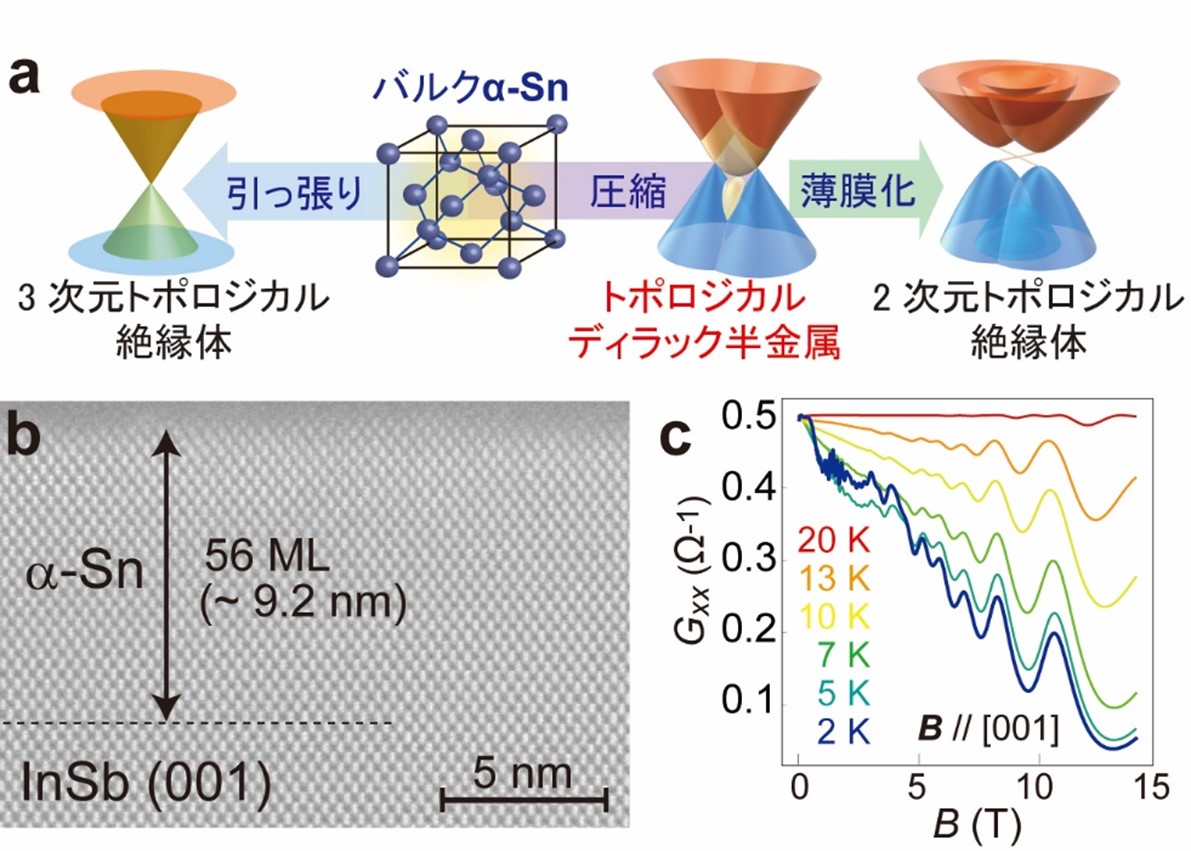
In topological materials (topological semimetals and insulators, etc.), "Dirac electrons", which possess a linear relationship (band dispersion) between energy and momentum (wavevector) like graphene, are responsible for bulk or surface electrical conduction. Dirac electrons have an extremely small effective mass and high mobility, so they are suitable for ultra-high-speed electronic devices. In addition, when a material has a strong spin-orbit interaction, the spin direction and momentum direction of Dirac electrons are strongly coupled, which is called "spin-momentum locking". As a result, the current due to the flow of Dirac electrons is accompanied by spin polarization, so the topological materials are promising for spintronics devices and quantum information devices.
In order to utilize topological materials in practical devices and integrated circuits, material quality and controllability, as well as compatibility with existing technologies are crucial. Topological materials that meet such requirements have not yet been established. We are pioneering the crystal growth and topological properties of topological Dirac semimetals, topological Weyl semimetals, topological insulators, and topological superconducting materials that are consistent with semiconductor technology. Beyond fundamental research on crystal growth and condensed matter physics, we aim to apply these attractive topological materials to future sensing devices, information processing, and storage technologies.